Self-healing and Self-repairing Materials. The past, present and future of Materials Science.
Self-healing and self-repairing materials represent a groundbreaking area of materials science that draws inspiration from nature's remarkable ability to heal and regenerate.
These innovative materials possess the capability to autonomously repair damage and restore functionality, leading to extended lifetimes, enhanced safety and reliability, and more sustainable use of resources.
Nature-inspired innovation plays a pivotal role in the development of self-healing materials, as researchers look to biological systems for insights into designing synthetic materials with similar regenerative properties.
The potential applications of self-healing materials are vast, spanning industries from construction and automotive to electronics and healthcare. By taking inspiration from the self-repair mechanisms found in living organisms, these materials offer a paradigm shift in how we design for damage tolerance and sustainability. This article will provide a comprehensive overview of the history, current state, key innovations, challenges, and future potential of self-healing and self-repairing materials, highlighting the critical role of nature-inspired innovation in advancing this exciting field.
Historical Context and Evolution
The concept of self-healing materials has its roots in early observations of natural systems that exhibit regenerative capabilities, such as the ability of skin to heal wounds or bone to repair fractures. Initial attempts to mimic this in synthetic materials focused primarily on polymers and composites in the 1980s and 1990s. A seminal breakthrough came in 2001 with the development of a polymer composite that could heal cracks via embedded microcapsules containing a healing agent. This pioneering work laid the foundation for further advances in the field.
Over the past two decades, research into self-healing materials has expanded rapidly, driven by the need for more durable, sustainable, and resilient materials across a wide range of applications. Advances in materials science, nanotechnology, and biomimetics have enabled the development of increasingly sophisticated self-healing systems that can respond to various types of damage and environmental stimuli.
The Current State of Self-Healing Materials
Today, self-healing materials encompass a diverse range of material classes including metals, ceramics, concrete, and polymers. At the macro scale, self-healing mechanisms include the release of healing agents from embedded microcapsules or vascular networks to fill and bond cracks. At the nanoscale, molecular mechanisms such as reversible bonding and chain rearrangement enable healing.
Exciting developments are occurring in self-healing electronics, where the ability to repair circuit damage could enable more sustainable and longer-lasting devices. Bio-inspired approaches that mimic natural structures like blood vasculature or skin are also being explored to create more robust and adaptive self-healing systems.
In the construction industry, self-healing concrete that can seal its cracks is being developed to improve the durability and sustainability of infrastructure. By incorporating bacteria or other healing agents, these materials can autonomously repair damage caused by weathering, loading, or chemical attack.
Key Developments and Innovations
Polymer composites reinforced with fibres or particles are one major area of innovation in self-healing materials. Biomimetic microvascular networks embedded within these composites enable continuous delivery of healing agents to damaged regions, allowing recovery of mechanical properties. This vascular approach mimics the healing process in biological tissues and can provide repeated healing of damage.
Another key development is the use of reversible dynamic bonds to create intrinsically self-healing polymers. These materials can heal via the reformation of hydrogen bonds, metal-ligand coordination, or dynamic covalent bonds when stimulated by heat, light, or other triggers. This molecular-level healing mechanism eliminates the need for additional healing agents and enables repeatable healing of damage.
The integration of self-healing capabilities with other functional properties such as actuation, sensing, or conductivity is another exciting frontier. For example, self-healing ionic conductors based on ion-dipole interactions have been developed for stretchable electronics. Self-healing liquid crystal elastomers have also been created that can actuate and change shape in response to stimuli.
Nature-inspired strategies like the use of living materials are also being explored for self-healing applications. Engineered living materials made from fungal mycelium have been shown to exhibit self-repair properties due to the regenerative capabilities of the living cells. This bio-hybrid approach opens up new possibilities for sustainable and adaptable self-healing materials.
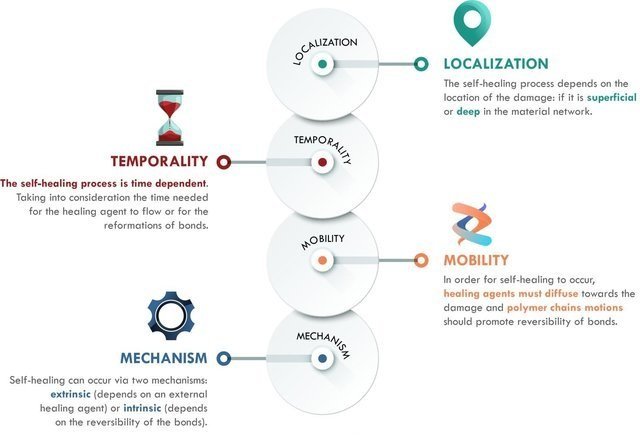
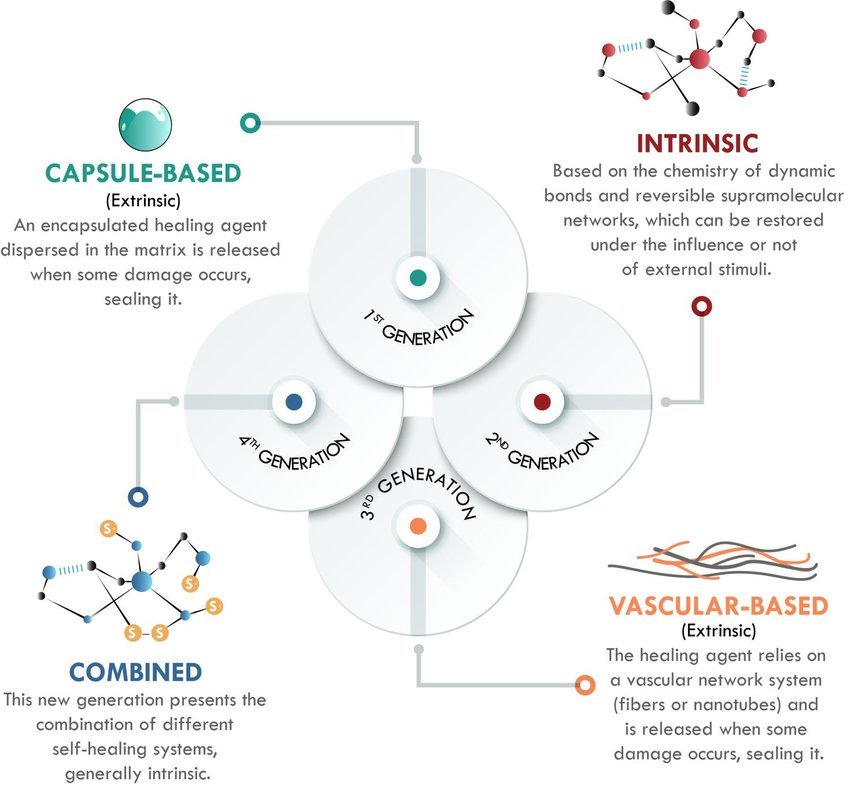
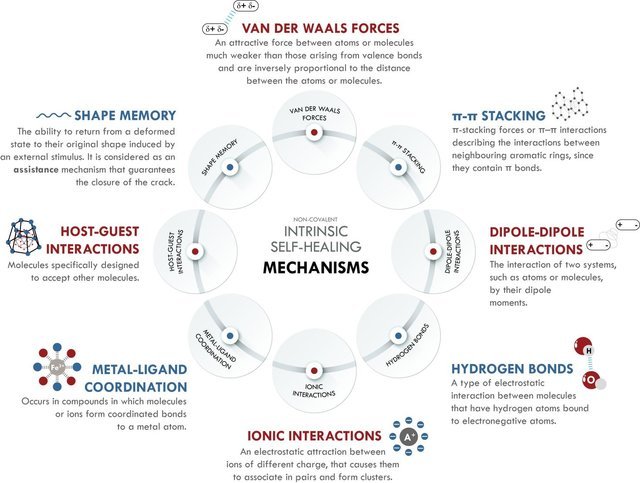
Challenges and Limitations
Despite significant progress, self-healing materials still face several challenges and limitations that must be addressed for widespread practical application. One key issue is the tradeoff between healing efficiency and other material properties like stiffness or toughness. Understanding the tradeoff between self-healing performance while maintaining the desired mechanical properties remains a challenge.
The long-term stability and functionality of healed regions is another concern, particularly for load-bearing applications. Ensuring that repaired areas can withstand repeated damage and maintain structural integrity over extended periods is crucial for the reliability of self-healing materials.
Scalability and cost are additional barriers to the commercialization of self-healing technologies. Many current self-healing systems rely on complex chemistries or processing methods that are difficult to scale up economically. Developing simpler, more affordable approaches to self-healing that can be easily integrated into existing manufacturing processes is an ongoing challenge.
Environmental and toxicological considerations are also important, especially for self-healing materials used in biomedical or eco-sensitive applications. Ensuring the biocompatibility and biodegradability of healing agents and avoiding the release of harmful substances are key priorities.
Future Directions and Potential
Looking ahead, the field of self-healing and self-repairing materials is poised for exciting advances that could transform the way we design and use materials in the future. By converging active, adaptive and autonomous properties, researchers aim to create fully "animate" materials that go beyond self-healing to emulate the dynamic, responsive and regenerative qualities of living systems.
In the short term, the biggest economic gains may come from increasing the "animacy" of materials used in civil infrastructure and industrial equipment. Self-healing concrete, asphalt, plastics and coatings could dramatically reduce maintenance costs while improving safety and efficiency. In medicine, animate materials may enable more precise, personalized and less invasive treatments that adapt to the body and actively promote healing.
In the long term, the development of programmable matter that can autonomously assemble, disassemble and reassemble into different functional shapes could revolutionize manufacturing and product design. Robotic materials and devices with built-in intelligence and resilience could be deployed in remote or hazardous environments. Data could be stored and processed in DNA, while everyday objects harvest energy from ambient sources.
Harnessing the power of nanotechnology, machine learning, and synthetic biology will be key to engineering materials with the multi-scale architectures and dynamic feedback loops found in living systems. Computational tools can accelerate the discovery of new self-healing chemistries, while also ensuring that sustainability is prioritized from the start.
Ultimately, animate materials may blur the line between non-living and living matter. Protocell research exploring the origins of life could lead to the emergence of novel life-like materials following different evolutionary pathways than biology. This would open a new era of material possibilities but also require careful consideration of the ethical, social and environmental implications.
The future of self-healing and animate materials will be shaped by the convergence of multiple scientific disciplines, from materials science and chemistry to biology, computer science and robotics. Fostering cross-disciplinary collaboration and public engagement will be essential to unlock the full potential of this exciting field while ensuring that risks are proactively managed and societal benefits are realised.
Explore the work of the Royal Society policy report for further insights.
Conclusions
Self-healing and self-repairing materials represent a remarkable example of how nature-inspired innovation can drive transformative advances in materials science and engineering. By mimicking the regenerative capabilities of living systems, these materials offer a pathway to creating more durable, adaptable, and sustainable products that can self-repair in response to damage or wear.
From construction and transportation to electronics and healthcare, the potential applications of self-healing materials are vast and far-reaching. As research in this field continues to accelerate, we can anticipate the development of even more advanced self-healing systems that blur the boundaries between living and non-living matter.
However, realising the full potential of self-healing materials will require ongoing investment in fundamental research, as well as close collaboration between academia and industry to translate breakthroughs into practical solutions. Addressing key challenges around performance, scalability, and sustainability will be critical to unlocking the commercial potential of these innovative materials.
Ultimately, the success of self-healing materials will depend not only on technological progress but also on societal acceptance and adoption. Engaging stakeholders across the value chain, from material producers to end-users, will be essential to drive the uptake of these technologies and maximize their positive impact.
As we look to the future, the field of self-healing materials offers a compelling vision of a world where our built environment and everyday objects are imbued with the resilience and adaptability of living systems. By embracing nature-inspired innovation and pushing the boundaries of materials science, we can create a more sustainable, resilient, and regenerative future for all.
Hi, we're Biomimicry Innovation Lab. We partner with founders and leaders to transform ideas into reality, drawing inspiration from transformative solutions found in nature. Our approach? Harnessing the latest scientific research with innovative tools to deliver solutions to complex challenges. Reach out for a virtual coffee to discuss ideas.
References:
Bandodkar, A.J., et al., 2019. Self-healing electronic materials for a smart and sustainable future. Advanced Materials, 31(39), p.1902869.
Binder, W.H., 2013. Self-healing polymers: from principles to applications. John Wiley & Sons.
Darabi, M.A., et al., 2020. Bioinspired self-healing materials: lessons from nature. Chemical Society Reviews, 49(15), pp.5140-5158.
De Belie, N., et al., 2018. A review of self-healing concrete for damage management of structures. Advanced Materials Interfaces, 5(17), p.1800074.
Diesendruck, C.E., et al., 2015. Self-healing polymer composites: mimicking nature to enhance performance. Bioinspiration & Biomimetics, 10(1), p.015003.
Hager, M.D., et al., 2010. Self-healing materials. Advanced Materials, 22(47), pp.5424-5430.
Hattori, G., et al., 2021. Self-healing materials and artificial intelligence: a game-changing combination. ACS Applied Materials & Interfaces, 13(1), pp.2011-2025.
Herbst, F., et al., 2013. Self-healing polymers via supramolecular forces. Polymer, 54(18), pp.4969-4984.
Li, Y., et al., 2019. Recent advances in intrinsic self-healing cementitious materials. Advanced Materials, 31(45), p.1901493.
Mauldin, T.C. and Kessler, M.R., 2010. Self-healing polymers and composites. International Materials Reviews, 55(6), pp.317-346.
Oh, J.Y., et al., 2019. Intrinsically stretchable and self-healable conductors based on ion-dipole interactions. Science, 366(6469), pp.1121-1126.
Scheiner, M., et al., 2016. Self-healing polymers. Polymer, 98, pp.389-416.
Thakur, V.K. and Kessler, M.R., 2015. Self-healing polymer nanocomposite materials: A review. Polymer, 69, pp.369-383.
Urdl, K., et al., 2017. Self-healing of densely crosslinked thermoset polymers—a critical review. Progress in Organic Coatings, 104, pp.232-249.
Wang, C., et al., 2018. Self-healing chemistry enables the stable operation of silicon microparticle anodes for high-energy lithium-ion batteries. Nature Chemistry, 10(6), pp.624-630.
Xiang, H.P., et al., 2020. Multifunctional self-healing ionogels with temperature-responsive, elastic, and self-adhesive properties as highly sensitive sensors and flexible electrodes. ACS Applied Materials & Interfaces, 12(7), pp.8555-8566.
Yang, Y. and Urban, M.W., 2013. Self-healing polymeric materials. Chemical Society Reviews, 42(17), pp.7446-7467.
Zhu, D.Y., et al., 2019. Biological and biomedical functions of self-healing polymers. Materials Today, 29, pp.114-126.
Zwaag, S.V.D., 2007. Self-healing materials: an alternative approach to 20 centuries of materials science. Springer Science & Business Media.